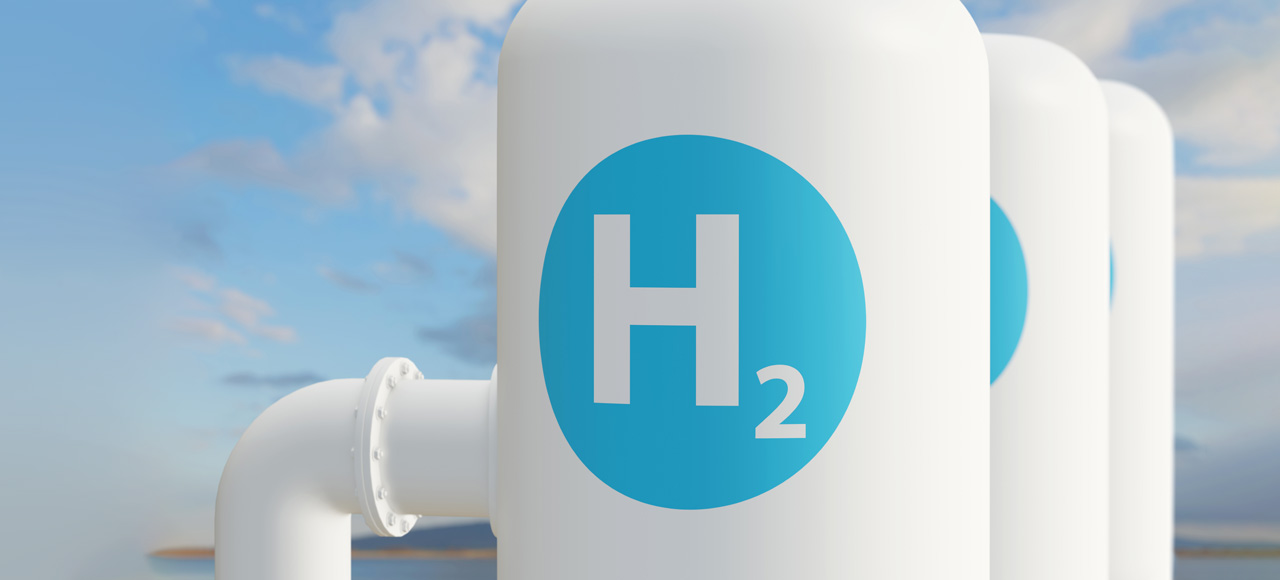
Challenges of Modeling Hydrogen in the U.S. Energy System
It will be impossible for the United States and other countries to achieve net-zero greenhouse gas emissions without improved and new clean energy sources. Hydrogen, which has been discussed for decades as a potential cornerstone technology, is thought by many to be critically important. Hydrogen is a clean burning fuel; can be manufactured from and used to produce low and zero-carbon energy; and is more suitable for use in certain carbon-intensive industrial applications than electrification.
More investment in research and development is required to bring H2 technologies to maturity. In addition, investment is needed in modeling how hydrogen can be integrated into national and international energy systems. Systems modeling is an essential predicate to developing policies for realizing hydrogen’s potential in the U.S. energy market.
This blog post summarizes the discussion from a March 24, 2022, webcast forum, organized by OnLocation, on the challenges of modeling hydrogen in the U.S. energy system. Sponsored by the Department of Energy’s Offices of Fossil Energy & Carbon Management (FECM) and Energy Efficiency and Renewable Energy (EERE), contributors included EIA, DOE, EPA, and leading energy system modeling teams. The forum, which drew an on-line audience of energy modelers and policy analysts, highlighted priorities for hydrogen production and utilization, and the challenges and approaches to modeling a hydrogen energy economy. The full set of presentations and the Executive Summary is available on the OnLocation website.
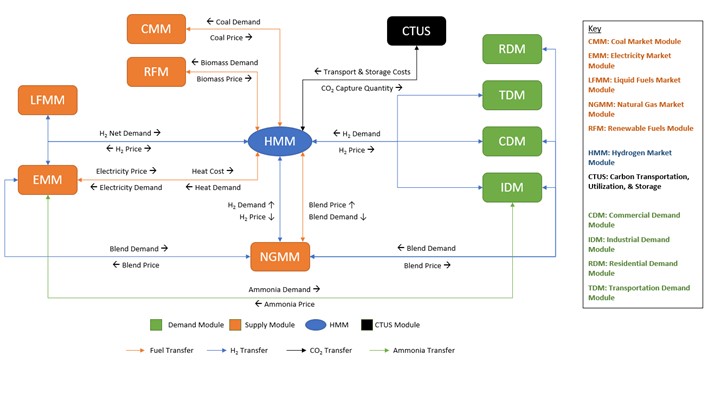
The Hydrogen Economy: Potential and Complexity
The hydrogen modeling and policy challenge is driven by the technology’s flexibility — there are multiple pathways for production and utilization. Currently most hydrogen is produced through steam methane reforming (SMR) of natural gas, a CO2-emitting process. Low and zero-carbon options include SMR linked to carbon capture and storage (CCS) and electrolysis using electricity produced from renewable and nuclear sources. Hydrogen can be transported by pipeline, tanker, or even truck and rail.
Hydrogen can be burned as a gas or as liquid ammonia, and it can be used to produce synthetic fuels in a low-carbon cycle linked to CO2 extracted from the atmosphere via direct air capture. The final fuel, in whatever form, can be used directly in combined and simple cycle generators to produce power; as a transportation fuel; stored for power generation; and used to produce heat in industrial applications which are difficult to electrify.
Hydrogen Modeling Challenges
Modeling a hydrogen future is a unique challenge because the analysis must incorporate a complete economy – production, transportation, storage, utilization, environmental and social impacts, and costs – for an energy system that for the most part does not exist. Current uses of hydrogen are primarily refining and chemicals, and 90 percent of hydrogen production is from SMR with no CCS. It is an enormous step to model the rest of the hydrogen pathways in the economy and the possible array of policies and incentives that could be used to develop and support the new market. Some of these challenges are described below.
- Fundamentals of cost and performance: Except for SMR, hydrogen technologies are universally pre-commercial, which makes modeling far more Significant uncertainties exist for many elements of the technology pathways, including production costs, many utilization costs, pipeline buildout, and the availability of storage sites. A complementary issue is that hydrogen technology data collection has been limited, although it is now ramping up.
- Market Structure: Hydrogen market structure is crucial to the analysis but is currently speculative. A major issue for policymakers is determining which incentives will produce the best market outcomes. Complicating domestic market issues is the international aspect. Because hydrogen can be transported in bulk via pipeline and tanker, and is much cheaper to produce via electrolysis in regions with low electricity costs, hydrogen could become an international commodity, a factor that must be accommodated in analyses.
- Electric Power: The power sector will be a key end-user of hydrogen, but interaction with the electric sector is complex. For example, there is a tradeoff between dedicated renewable energy for hydrogen electrolysis vs. using renewable power directly for grid decarbonization – which application provides more carbon bang for the buck? Another issue is storage, where hydrogen provides a different value proposition than battery storage. In general, modeling hydrogen requires evaluating production and consumption using seasonal or more granular, even hourly, time intervals.
- Transportation: Medium and heavy-duty trucks are early candidates for hydrogen conversion. However, hydrogen’s competitive value compared to battery drive depends on the duty cycle of the vehicle, and the time available for recharging. Obtaining updated data on truck technology is a priority. Additional transportation sector roles for hydrogen may include the off-road (e.g., forklifts) and marine sectors (where hydrogen-derived ammonia might replace bunker fuel).
- Environmental and Social Impacts: There are potential environmental effects from the generation, transport, storage, and use of hydrogen. Hydrogen leakage is an area of concern and can react with other gases causing air pollution and greenhouse gas concerns. Water scarcity impacts are unclear. Environmental justice concerns related to hydrogen will also be important to address in the evaluation of energy transition pathways.
- Policy and Incentives: Regulatory policy for hydrogen is in its infancy. Consequently, modeling has much to contribute to policy development, but also faces the challenge of creating and analyzing new and unique scenarios. To cite just one issue, an incentive regime will have to differentiate between the various flavors of hydrogen in terms of the carbon intensity of the production process and the carbon reduction payoff from different end-uses. This is not straightforward; for example, SMR has fixed CO2 intensity, while electrolysis depends on the CO2 intensity of the electricity grid.
Addressing the Modeling Challenge
The Bipartisan Infrastructure Law requires EIA to develop enhanced tools for modeling the hydrogen economy. OnLocation, which for decades has been the prime contractor for the National Energy Modeling System (NEMS), is assisting EIA, FECM, and EERE with developing the model’s new Hydrogen Market Module (HMM). Other NEMS components will be updated to incorporate hydrogen, including:
- Electricity Market Module: H2 as a fuel for power generation; electrolysis demand for electricity; hydrogen for seasonal storage and reliability.
- Natural Gas Market Module: Blended hydrogen in natural gas pipelines.
- Transportation Module: Current modeling focus is hydrogen as a fuel for heavy-duty vehicles.
- Industrial Module: Hydrogen as an energy source for the hard-to-electrify steel, chemical, and cement sectors.
- Liquid Fuels Market Module: Hydrogen used in refining.
- Commercial Demand Module: Niche combined heat and power uses
- Modeling of a dedicated pipeline transportation network and large-scale storage
OnLocation is also assisting EIA and DOE with incorporating other clean energy technologies into NEMS, such as enhanced representation of carbon capture, transport, utilization and storage (CTUS-NEMS). The linkages between CTUS, HMM, and the other elements of NEMS are shown in the accompanying graphic (Figure 1).